Phase Change Materials: Is it the Future of RF Switch Technology?
A phase-change-material acts like glass or metal after an appropriate thermal pulse and can be used for fantastic RF switching. But how does it compare to RF silicon-on-insulator technology?
Phase change materials (PCMs), which were formerly used in memory applications, are now finding new uses in RF circuits as technology advances.
Is it good enough to replace RFSOI, the 800-pound switch gorilla that has dominated RF front-end circuits for more than a decade? With new emerging standards, PCM could be just what the industry needs. PCM switches overcome some of the limitations of RFSOI for mmWave applications while also introducing new ones.
In this essay, we will go deep into PCM technology for RF and examine how it compares to existing switch technologies such as RFSOI.
Here is what we will cover:
PCM and Intel’s X-Point Technology
How Phase Change Materials Work
PCM for RF Switch Technology
Measuring up against RFSOI
Read time: 13 mins
This post is long, and is best read online due to email size limitations.
PCM and X-Point Technology
If the term phase change material sounds familiar, it is because it was marketed as an alternative to MOS-based Dynamic Random Access Memory (DRAM) by a joint venture between Intel and Micron in 2016. Intel introduced the Optane family of memory and SSDs based on 3D X-point technology, which they claimed were not phase change memory, when it actually was.
The unique aspect of 3D X-point technology is that no transistors were used to build memory. To develop non-volatile memory, it used a unique substance known as a chalcogenide, an alloy of a type of metalloids with characteristics similar to both metals and nonmetals, instead of a transistor. When heated or cooled, these materials transition from amorphous to crystalline, and vice versa. They shift between phases. The change in electrical characteristics is utilized to store a 0 or 1 in a method that lasts even when no power is applied to it.
Optane SSDs using X-point technology were extremely durable and fast, but came at a hefty cost. If money wasn't a problem, these were the best SSDs you could buy. If you believe that PCM transformed the computing world, you are correct. It did it in such a way that a new class of materials could significantly advance technology as ancient as memory. Here's the kicker: Intel discontinued the commercial use of the technology in 2021. Why? The economics did not work out.
PCM is re-emerging in RF switches for the next generation of communication technologies. In 2024 alone, IEEE Microwave Magazine published two articles solely about PCM. Tower Semiconductor has integrated it into its technological backends. The faraway drum of PCM technology draws closer, this time aimed at RF applications.
If you are new to RF switches, please see an earlier post below on the history of RF switch technology to put the contents of this article in context. It also introduces all of the RF switch-related terminology that will be used throughout this post.
How Phase Change Materials Work
Phase change materials are compounds made up of metalloids (light green on the periodic table) and group 16 elements known as chalcogens (red square) to generate chalcogenides. Tellurium (Te) is the only stable metalloid that also functions as a chalcogen. It is frequently alloyed with tin (Sb) and/or germanium (Ge) to produce a class of materials that change state when heated to particular temperatures.
There are two states in which PCMs exist:
Amorphous: The constituent atoms are in disorderly state where no electrical conduction happens.
Crystalline: The constituent atoms are arranged in a orderly crystal lattice through which current can flow with low resistance.
A thermal pulse applied to a PCM causes it to shift state. In an integrated circuit implementation, pulses are produced by putting a voltage across a thin film resistor, causing current flow and a local temperature rise. When the temperature hits recrystallization temperature (Tc) and is maintained for around a microsecond, the amorphous PCM molecules swiftly align into a crystalline structure in a few tens of nanoseconds. At this time, the voltage can be removed from the material, and it remains in the on-state, operating as a resistor.
When a higher voltage is applied to push enough current to reach an even higher melting temperature (Tm) and then removed, the crystalline structure melts back into an amorphous state, passing through the recrystallization temperature too quickly to form crystals again. At this time, the material becomes amorphous and non-conducting, providing a significant resistance to the flow of current.
A popular PCM is Ge2-Sb2-Te5, which is a 2:2:5 ratio of Germanium, Antimony, and Tellurium, often known as GST. GeTe is also a popular PCM material for RF switches. The temperatures required for phase change vary according on the material composition utilized, and are typically the "secret sauce" of PCM technology.
If all of this sounds fantastic, you should also be amazed by rewritable DVDs, which use GST or a similar alloy using Indium or Silver instead of Germanium [3]. A blank DVD is the crystalline version of the chalcogenide, and instead of voltage, a write laser is used to heat certain places and generate amorphous pits in the CD. To erase the DVD, a low power laser is utilized to recrystallize the material and return it to its original blank condition.
PCM for RF Switches
The idea of PCM for RF switches is not new. As early as the 2000s, when it was found that PCM can be integrated in a standard CMOS manufacturing process and used for digital memory, researchers started investigating their use for RF switches. All through the 2010s, various entities such as Carnegie Mellon University, Northrup Grumman, HRL Labs, University of Waterloo and University de Limoges worked on developing this technology for RF applications. They achieved Ron-Coff products of under 20 femtoseconds, which is two times better than the state-of-the-art RFSOI FET switch in existence.
While all entities made significant progress on the technology over the years, they all gave eventually gave up on commercializing the technology due to two reasons:
Power handling: The crystalline on-state of the PCM switch is the material's stable state, and it can withstand high power levels (>35 dBm, or >12 volts) without breaking down. The problem is in the switch's (amorphous) off-state, where an increase in RF power provides enough heating to partially recrystallize the PCM, eventually causing the switch to break down. Initially, these switches could only withstand 2 volts, but later modifications allowed for up to 4 volts (~25 dBm @ 50 ohms) across them.
The astute reader may observe that RFSOI switches can handle 3-4 volts of off-state voltage. To achieve stronger voltage handling, switches are typically stacked in FET switches. The same technique can be done using PCM switches, but their low-loss advantage disappears quickly, leaving no reason to abandon FET-based switches.Endurance: A switch must be entirely functional even after a billion actuations. However, early PCM-based RF switches had a problem with on-resistance drift. This means that after 100 million actuations, the switch's on-state resistance increased by nearly 70%—an unacceptable value. Ideally, we want the switch's on-resistance to remain constant throughout its life.
Today, Tower Semiconductor is the only foundry to offer a commercial version of PCM technology integrated into RFSOI, BiCMOS and Power MOS technology backends. As we will see, they have overcome a lot of the issues that have plagued PCM switches in the past to create what seems like a true competitor to RFSOI.
The integration of PCM switches with BiCMOS technology is especially noteworthy. SiGe heterojunction bipolar transistors (HBTs) are exceptional RF devices with high output impedance, low noise, and excellent linearity. They are commonly employed in high-performance RF circuits like phased arrays, but switch performance has traditionally been the bottleneck. The bulk silicon implementation of BiCMOS technology has traditionally hampered the RF performance of FET switches due to parasitic effects. PCM switches in BiCMOS technology now offer both low-loss switches and high-performance RF transistors. The circuits that result from this approach should be interesting.
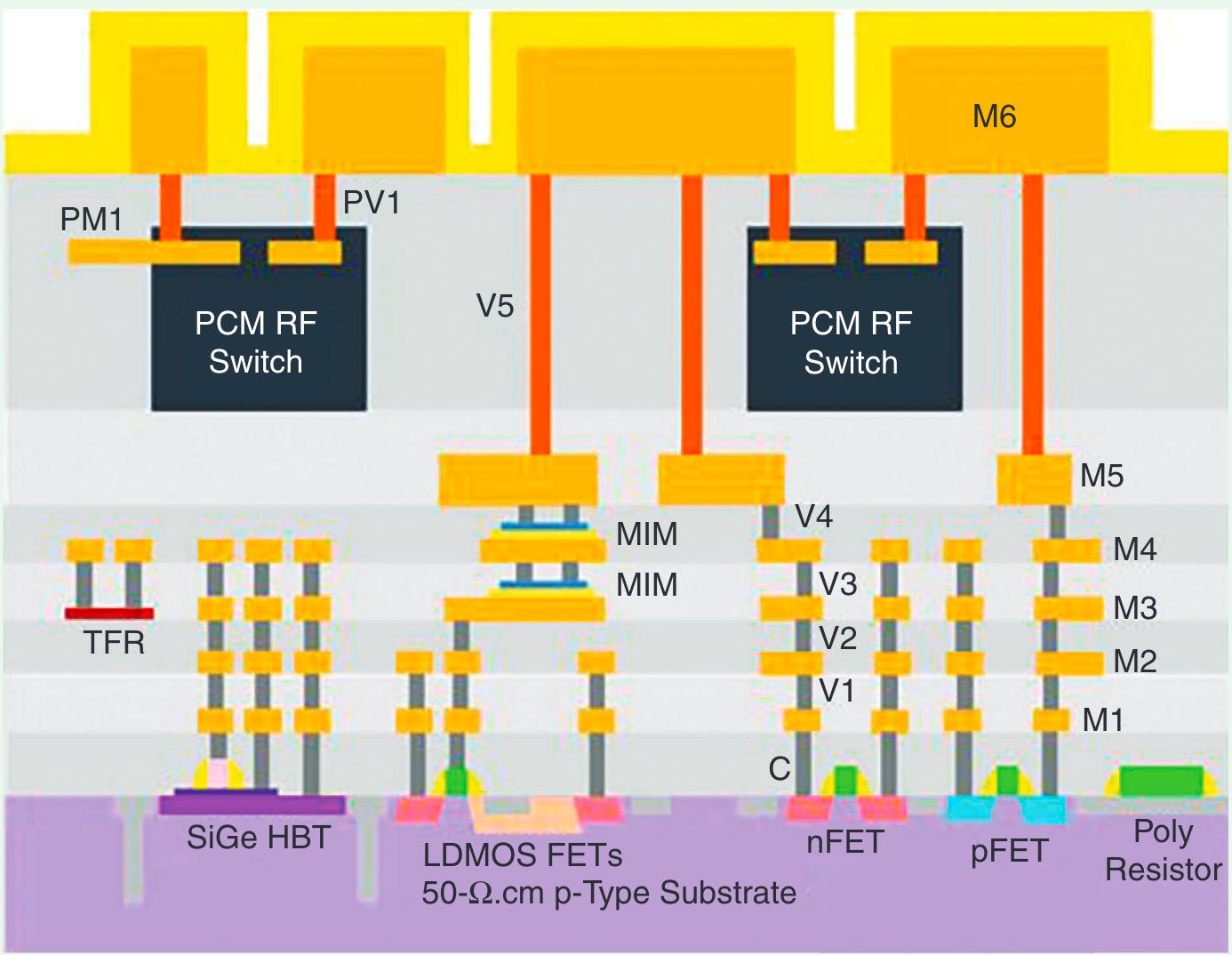
Measuring Up Against RFSOI Switches
Apart from certain niche applications, RFSOI has dominated switch technology over the last 10-15 years. When a new technology emerges, it should be measured up against RFSOI switches to determine if the technology will be here to stay. As the authors in [1] describe, we can evaluate PCM-based switches against several criteria:
Ron-Coff: This primary figure of merit is what drives all of RF switch development. In this regard, PCM-based switches provide exceptional performance and is the primary reason these switches are being considered for mm-wave frequencies and beyond. Tower’s 10 and 20 micron wide PCM switches provide <20 fs up to 125°C. In comparison to other switching technologies, this represents an improvement that is several multiples lower than what RFSOI is capable of. Such low-loss performance opens up the doors to high throw count switches at high frequencies with a small layout footprint.
Image credit: Tower Semiconductor’s rather generous marketing numbers for PCM RF switches. Power handling: The asymmetric voltage breakdown in the on and off states has been a problem for both RFSOI and PCM switches. The on-state power handling in RFSOI switches is dictated by the metal backend's electromigration rules, but in PCM switches, it is determined by the thermal capabilities of the stable crystalline structure. As a result, power handling is generally substantially higher in the on state. In the off-state, RFSOI switches are subject to soft breakdown when the power level increases due to the floating body effect, where stored charges in the silicon layer exit through the transistor drain due to impact ionization. In the off-state, PCM switches are subject to recrystallization at high RF power, as we discussed earlier. In the case of a multi-throw switch, the lower of the two breakdown voltages is always the limiting factor.
By carefully engineering the composition of the PCM layer, Tower’s PCM switch shows symmetric breakdown voltages of 10 volts in the on- and off-state. This is a significant number because you would need to stack about three RFSOI switches to achieve the same breakdown voltage, which means that the equivalent on-resistance would increase by 3x as well. This is a dramatic improvement in the performance of RF switch technology. It remains to be seen how well this number holds up versus temperature. In [1], Tower hints that PCM switches can support at least 7 volts at 125°C which is still pretty good. In terms of linearity, PCM switches appear to be much more linear than RFSOI switches too.Endurance: Tower’s reliability testing results show that the on-resistance drifts 7% at 1 billion actuations, and 30% at 10 billion actuations. While this is a significant improvement from earlier implementations of PCM switches, it remains to be seen if this is an acceptable level of performance for high frequency applications, which are often deployed to have long lifetimes in the market. It also depends if the application involves low-switching rates where the PCM switches will not reach their end of actuation life quickly.
Switching speed: Semiconductor based switches have the fastest actuation speeds typically in tens of nanoseconds, while MEMS switches are slow at tens of microseconds. The PCM switch has a switching time somewhere in between FET switches and MEMS, at about 0.7 microseconds. The use of PCM switches will again depend on whether the application can handle these switching times.
Power consumption: PCM switches only consume power during the switch actuation event, whereas RFSOI switches constantly consume power to hold the switch in the off-state1. During the actuation event, up to 100mA of current can flow through the switch with an actuation voltage of 3-10 volts, depending on the width of the switch2. While it is nice that power is consumed only during actuations, the large current spike and requirement of up to 10 volts on-chip is cause for concern in the design of monolithic biasing circuits for commercial applications.
Integration, at-scale manufacturing and cost: Since PCM as a base technology has been commercialized in memory applications already, the integration of these switches in a standard CMOS line is a solved problem for the most part. As a result, manufacturing these switches at-scale should also be doable although the question of yield is still publicly unknown in the context of RF PCM switches.
The cost-adder per wafer for including PCM switches plays a significant role in whether these switches are adopted for RF applications. As we've seen with Intel Optane memory and 3D X-point technology, economies of scale must be considered if these PCM switches are to substitute RFSOI. This did not happen with memory. If this technology is expensive, it will find a place in high-end applications with a high enough ASP to cover the cost (Intel Optane is still used on the server side). RFSOI switches will continue to dominate at frequencies below 6 GHz due to their adequate performance. PCM switches may find a home in higher frequency applications such as satellite communications, phased arrays, wireless backhaul, automotive, and future 6G standards.
We will leave this post with an extensive comparison of RF PCM switches with other technologies from [1] that will require quite a bit of staring at to really soak in the numbers. I also highly recommend reading the entire article. It covers a lot of detail.
References
[1] N. El-Hinnawy, G. Slovin, K. Moen, C. Masse, and D. Howard, “A Tale of Two Phases: An Overview of Phase-Change Material RF Switch Technology: Sub-20-fs R ON*C OFF RF Switch Technology for the Mm-Wave and 5G/6G Revolutions,” IEEE Microwave, vol. 25, no. 8, pp. 57–76, Aug. 2024.
[2] T. Singh, N. K. Khaira, M. Repeta, and R. R. Mansour, “Phase-Change RF Devices for Future Communications: Phase-Change Materials and Devices for Reconfigurable RF Front-Ends: State-of-the-Art and Future Perspectives,” IEEE Microwave, vol. 25, no. 2, pp. 18–38, Feb. 2024.
[3] A. V. Kolobov, P. Fons, J. Tominaga, and T. Uruga, “Why DVDs work the way they do: The nanometer-scale mechanism of phase change in Ge–Sb–Te alloys,” Journal of Non-Crystalline Solids, vol. 352, no. 9–20, pp. 1612–1615, Jun. 2006.
[4] T. Singh and R. R. Mansour, "Characterization, Optimization, and Fabrication of Phase Change Material Germanium Telluride Based Miniaturized DC–67 GHz RF Switches," in IEEE Transactions on Microwave Theory and Techniques, vol. 67, no. 8, pp. 3237-3250, Aug. 2019.
If you like this post, please click ❤️ on Substack, and tell someone if you like it.
If you enjoyed this issue, reply to the email and let me know your thoughts, or leave a comment on this post.
We have a community of RF professionals, enthusiasts and students in our Discord server where we chat all things RF. Join us!
The views, thoughts, and opinions expressed in this newsletter are solely mine; they do not reflect the views or positions of my employer or any entities I am affiliated with. The content provided is for informational purposes only and does not constitute professional or investment advice.
Gate induced drain leakage (GIDL) causes a constant gate current when the switch is held in the off-state with a certain negative voltage at the gate. The power draw must be supported by the charge pumps biasing the switch.
Wider PCM switches need more actuation voltage, compared to narrow switches.